Introduction
Sprinting is one of the most explosive and physically demanding activities in sports. Whether it’s a 100-meter race or a short burst on the football field, a sprint requires maximum effort in a short period. But what actually happens inside the body during a sprint? From energy production to muscle activation, sprinting is a complex physiological process involving multiple systems working together to generate power, speed, and efficiency. Let’s break down the mechanics and physiology behind this high-intensity movement.
The Phases of a Sprint
A sprint can be divided into four primary phases: the reaction phase, acceleration phase, maximum velocity phase, and deceleration phase. Each of these stages has unique physiological and biomechanical characteristics.
Reaction Phase
The sprint starts before movement even begins. As soon as the starting signal sounds, the brain sends rapid signals through the nervous system to activate the muscles. The quicker the reaction, the better the start. Elite sprinters have exceptionally fast reaction times, often under 0.15 seconds.
Acceleration Phase
In this phase, the athlete pushes explosively against the ground, generating forward momentum. The key to a strong acceleration is force production—stronger leg drive equals a faster start. Sprinters rely on the ATP-PC energy system here, which provides immediate energy but lasts only about 10 seconds.
Maximum Velocity Phase
At peak speed, the body reaches its most efficient biomechanical state. The neuromuscular system fires at its highest capacity, utilizing fast-twitch muscle fibers (Type II) to generate rapid, powerful contractions. Stride length and stride frequency are optimized to maintain velocity.
Deceleration Phase
Even the fastest sprinters begin to slow down due to fatigue. Energy depletion and the accumulation of metabolic byproducts, such as lactate, lead to reduced muscle power. Proper training can delay this phase, allowing an athlete to maintain top speed longer.
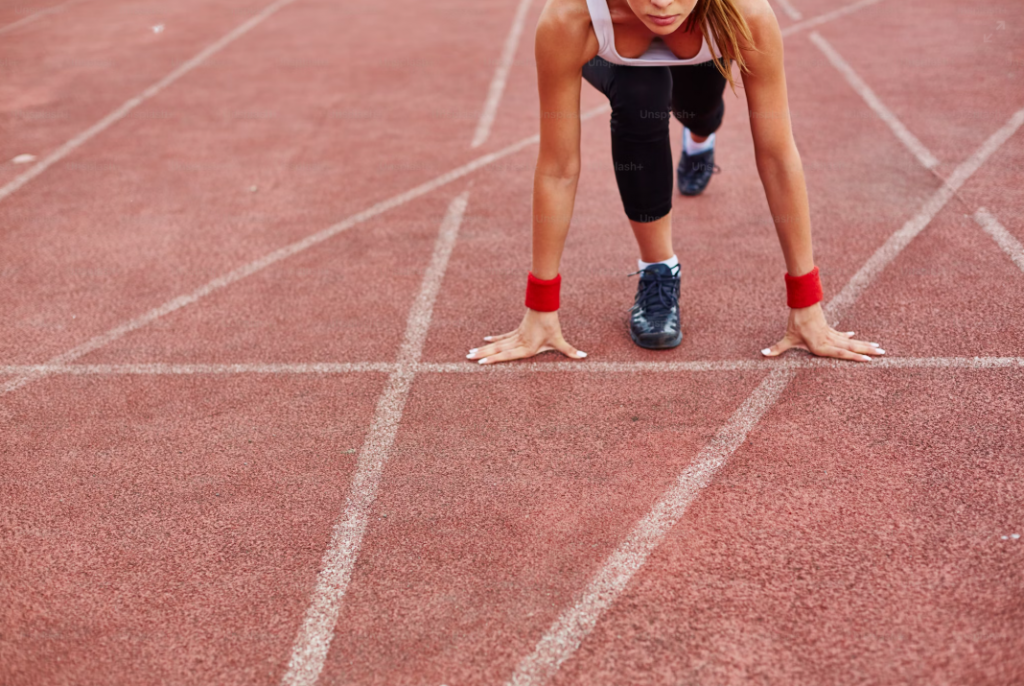
Energy Systems at Play in Sprinting
Sprinting relies on three primary energy systems, but one dominates the process.
ATP-PC System (Immediate Energy System)
This system provides energy for short bursts of activity, lasting up to 10 seconds. ATP (adenosine triphosphate) is stored in muscles and is broken down rapidly to fuel explosive movements.
Glycolytic System (Short-Term Energy System)
When the ATP-PC system depletes, the body shifts to anaerobic glycolysis, which breaks down glucose for energy. This system provides energy for up to 30 seconds but leads to the buildup of lactic acid.
Aerobic System (Minimal Role in Sprinting)
While essential for endurance sports, the aerobic system plays a minor role in sprinting, only aiding recovery between sprint repetitions.
Neuromuscular Involvement in Sprinting
Sprinting requires rapid muscle contractions, which depend on the neuromuscular system.
Fast-Twitch Muscle Fibers (Type II Fibers)
These fibers generate the power needed for sprinting. Unlike slow-twitch fibers (used in endurance activities), fast-twitch fibers contract quickly but fatigue rapidly.
Motor Unit Recruitment
The nervous system recruits motor units based on force demands. In sprinting, the body prioritizes high-threshold motor units, which produce the most force.
The Role of Biomechanics in Sprinting Efficiency
Proper biomechanics improve sprint speed and efficiency.
Stride Length vs. Stride Frequency
An optimal balance between stride length (how far each step covers) and stride frequency (how fast each step occurs) maximizes speed.
Ground Reaction Forces
Applying force efficiently into the ground generates forward propulsion. Elite sprinters optimize their force application with each step.
Joint Angles and Posture
Proper knee, hip, and ankle alignment reduces energy leaks and enhances sprinting mechanics.
The Impact of Sprinting on the Body
Sprinting challenges multiple physiological systems.
Cardiovascular Responses
Although short, sprints cause rapid heart rate spikes, increasing cardiovascular efficiency over time.
Muscular and Skeletal Stress
The high impact of sprinting places stress on muscles, tendons, and joints, making recovery crucial.
Training Strategies to Enhance Sprint Performance
Improving sprint performance requires targeted training.
Strength Training for Explosiveness
Exercises like squats, deadlifts, and plyometrics build the power needed for rapid acceleration.
Sprint Drills for Speed Development
Drills such as resisted sprints and sled pulls enhance technique and force application.
Recovery Techniques for Optimal Performance
Proper nutrition, hydration, and sleep support sprint recovery and performance.
Conclusion
Sprinting is more than just running fast—it’s a finely tuned process involving energy systems, neuromuscular coordination, and biomechanical efficiency. Understanding the physiology of a sprint can help athletes train smarter and run faster.